COVID-19 vaccine: What’s RNA research got to do with it?
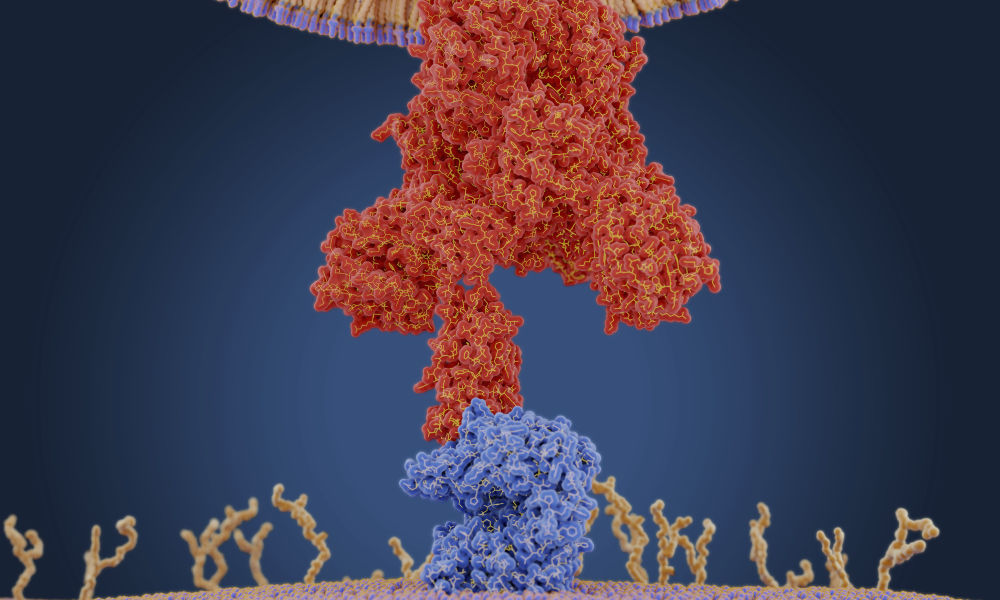
The US Food and Drug Administration recently approved emergency use authorization for a COVID-19 vaccine developed by Pfizer and the German pharmaceutical company BioNTech.
The vaccine made history not only because it reported a 95 percent efficacy rate at preventing COVID-19 in clinical trials, but because it is the first vaccine ever approved by the FDA for human use that is based on RNA technology.
“The development of RNA vaccines is a great boon to the future of treating infectious diseases,” says Lynne Maquat, the J. Lowell Orbison Distinguished Service Alumni Professor in biochemistry and biophysics, oncology, and pediatrics at Rochester and the director of Rochester’s Center for RNA Biology.
COVID-19, short for “coronavirus disease 2019,” is caused by the novel coronavirus SARS-CoV-2. Like many other viruses, SARS-CoV-2 is an RNA virus. This means that, unlike in humans and other mammals, the genetic material for SARS-CoV-2 is encoded in ribonucleic acid (RNA). The viral RNA is sneaky: its features cause the protein synthesis machinery in humans to mistake it for RNA produced by our own DNA.
For that reason, several of the leading COVID-19 vaccines and treatments are based on RNA technology.
A contingent of researchers at the University of Rochester study the RNA of viruses to better understand how RNAs work and how they are involved in diseases. This RNA research provides an important foundation for developing vaccines and other drugs and therapeutics to disrupt the virus and stop infections.
“Understanding RNA structure and function helps us understand how to throw a therapeutic wrench into what the COVID-19 RNA does—make new virus that can infect more of our cells and also the cells of other human beings,” Maquat says.
In the past few decades, as scientists came to realize that genetic material is largely regulated by the RNA it encodes, that most of our DNA produces RNA, and that RNA is not only a target but also a tool for disease therapies, “the RNA research world has exploded,” Maquat says. “The University of Rochester understood this.”
In 2007, Maquat founded The Center for RNA Biology as a means of conducting interdisciplinary research in the function, structure, and processing of RNAs. The Center involves researchers from both the River Campus and the Medical Center, combining expertise in biology, chemistry, engineering, neurology, and pharmacology.
“Our strength as a university is our diversity of research expertise, combined with our highly collaborative nature,” says Dragony Fu, an associate professor of biology on the River Campus and a member of the Center for RNA Biology. “We are surrounded by outstanding researchers who enhance our understanding of RNA biology, and a medical center that provides a translational aspect where the knowledge gained from RNA biology can be applied for therapeutics.”
How does RNA relate to disease?
A graphic created by the New York Times illustrates how the coronavirus that causes COVID-19 enters the body through the nose, mouth, or eyes and attaches to our cells. Once the virus is inside our cells, it releases its RNA. Our hijacked cells serve as virus factories, reading the virus’s RNA and making long viral proteins to compromise the immune system. The virus assembles new copies of itself and spreads to more parts of the body and—by way of saliva, sweat, and other bodily fluids—to other humans.
“Once the virus is in our cells, the entire process of infection and re-infection depends on the viral RNA,” Maquat says.
One of the reasons viruses are such a challenge is that they change and mutate in response to drugs.
That means novel virus treatments and vaccines have to be created each time a new strain of virus presents itself. Armed with innovative research on the fundamentals of RNA, scientists are better able to develop and test therapeutics that directly target the RNAs and processes critical to a virus’s life cycle.
How do RNA vaccines work?
Traditional vaccines against viruses like influenza inject inactivated virus proteins called antigens. The antigens stimulate the body’s immune system to recognize the specific virus and produce antibodies in response, with the hope that these antibodies will fight against future virus infection.
RNA-based vaccines—such as those developed by Pfizer/BioNTech and American biotechnology company Moderna—do not introduce an antigen, but instead inject a short sequence of synthetic messenger RNA (mRNA) that is enclosed in a specially engineered lipid nanoparticle. This mRNA provides cells with instructions to produce the virus antigen themselves.
Once the mRNA from a vaccine is in our body, for example, it “instructs” the protein synthesis machinery in our cells, which normally generates proteins from the mRNAs that derive from our genes, to produce a piece of the SARS-CoV-2 virus spike protein. Since the SARS-CoV-2 virus spike protein is foreign to our bodies, our bodies will then make antibodies that inactivate the protein.
“Should the virus enter our body from an infected person, these antibodies will bind to and inactivate the virus by binding to its spike proteins, which coat the outside of the viral capsule,” Maquat says.
An RNA-based vaccine therefore acts as a code to instruct our human cells to make many copies of the virus protein which, as a consequence, creates antibodies resulting in an immune response.
Unlike more traditional vaccines, RNA-based vaccines are also beneficial in that they eliminate the need to work with the actual virus.
“Working with a live virus is costly and very involved, requiring that researchers use special biosafety laboratories and wear bulky personal protective equipment so that the virus is ‘biocontained,’ and no one gets infected,” Maquat says.
Developing a vaccine from a live virus additionally takes much longer than generating an mRNA-based vaccine, but “no one should think the process is simple,” Maquat says of the Pfizer/BioNTech vaccine. “Since it is the first of its kind, a lot had to be worked out.”
What does RNA stand for?
RNA stands for ribonucleic acid.
What is RNA?
RNA delivers the genetic instructions contained in DNA to the rest of the cell.
What does Covid stand for?
Covid-19 stands for “coronavirus disease 2019.”
How is Rochester’s RNA research applicable to COVID-19?
Researchers Douglas Anderson, Dragony Fu, and Lynne Maquat are among the scientists at the University of Rochester who study the RNA of viruses to better understand how RNAs work and how they are involved in diseases. (University of Rochester photos / Matt Wittmeyer / J. Adam Fenster)
Maquat has been studying RNA since 1972 and was part of the earliest wave of scientists to realize the important role RNA plays in human health and disease.
Our cells have a number of ways to combat viruses in what can be viewed as an “arms race” between host and virus. One of the weapons in our cells’ arsenal is an RNA surveillance mechanism Maquat discovered called nonsense-mediated mRNA decay (NMD).
“Nonsense-mediated mRNA decay protects us from many genetic mutations that could cause disease if NMD were not active to destroy the RNA harbouring the mutation,” she says.
Maquat’s discovery has contributed to the development of drug therapies for genetic disorders such as cystic fibrosis, and may be useful in developing treatments for coronavirus.
“NMD also helps us combat viral infections, which is why many viruses either inhibit or evade NMD,” she adds. “The genome of the virus COVID-19 is a positive-sense, single-stranded RNA. It is well known that other positive-sense, single-stranded RNA viruses evade NMD by having RNA structures that prevent NMD from degrading viral RNAs.”
Maquat’s lab has been collaborating with a lab at Harvard University to test how viral proteins can inhibit the NMD machinery.
Their recent work is focused on the SARS-CoV-2 structural protein called N. Lab experiments and data sets from infected human cells indicate this virus is unusual because it does not inhibit the NMD pathway that regulates many of our genes and some of the virus’s genes. Instead, the virus N protein seems to promote the pathway.
“SARS-CoV-2 reproduces its RNA genome with much higher efficiency than other pathogenic human viruses,” Maquat says. “Maybe there is a connection there; time will tell.”
In the Department of Biology, Fu and Jack Werren, the Nathaniel and Helen Wisch Professor of Biology, received expedited funding awards from the National Science Foundation to apply their expertise in cellular and evolutionary biology to research proteins involved in infections from COVID-19. The funding was part of the NSF’s Rapid Response Research (RAPID) program to mobilize funding for high priority projects.
Werren’s research will be important in ameliorating some of the potential side effects of COVID-19 infections, including blood clots and heart diseases, while Fu’s research will provide insight into the potential effects of viral infection on human cell metabolism.
“Our research will provide insight into the potential effects of viral infection on host cellular processes,” Fu says. “Identifying which cell functions are affected by the virus could help lessen some of the negative effects caused by COVID-19.”
Douglas Anderson, an assistant professor of medicine in the Aab Cardiovascular Research Institute and a member of the Center for RNA Biology, studies how RNA mutations can give rise to human disease and has found that alternative therapeutics, such as the gene-editing technology CRISPR, may additionally “usher in a new approach to how we target and combat infectious diseases,” he says.
For the past few years, Anderson’s lab has developed tools and delivery systems that use the RNA-targeting CRISPR-Cas13 to treat human genetic diseases that affect muscle function. CRISPR-Cas13 is like a molecular pair of scissors that can target specific RNAs for degradation, using small, programmable guide RNAs.
When the health crisis first became apparent in Wuhan, China, researchers in Anderson’s lab turned their focus toward developing a CRISPR-Cas13 therapeutic aimed at SARS-CoV-2. Applying the knowledge already available about coronavirus RNA replication, they designed single CRISPR guide RNAs capable of targeting every viral RNA that is made within a SARS-CoV-2 infected cell. Using a novel cloning method developed in Anderson’s lab, multiple CRISPR guide-RNAs could be packaged into a single therapeutic vector (a genetically engineered carrier) to target numerous viral RNA sites simultaneously. The multi-pronged targeting strategy could be used as a therapy to safeguard against virus-induced cell toxicity and prevent ‘escape’ of viruses which may have undergone mutation.
“Infectious viruses and pandemics seemingly come out of nowhere, which has made it hard to rapidly develop and screen traditional small molecule therapeutics or vaccines,” Anderson says. “There is a clear need to develop alternative targeted therapeutics, such as CRISPR-Cas13, which have the ability to be rapidly reprogrammed to target new emerging pandemics.”
While many new treatments for the novel coronavirus are being considered, there is one thing that is certain, Maquat says: “Targeting RNA, or the proteins it produces, is essential for therapeutically combatting this disease.”
What role will RNA play in the future of vaccines and disease treatments?
Most people living in the United States today have only read about the 1918 flu pandemic and the relatively recent RNA viruses, such as Ebola or Zika, that are seen largely in other countries.
“RNA treatments will most likely be a wave of the future for these and other emerging diseases,” Maquat says. “Epidemiologists know new infectious pathogens are coming given how small the world has become with international travel, including to and from places where humans and animals are in close contact.”
Bats, in particular, are reservoirs for viruses. Many bat species are able to live with viruses without experiencing ill effects, given the bats’ unusual physiology. If these bat viruses mutate so they become capable of infecting humans, however, there will be new diseases, Maquat says.
“It is just a matter of when this will happen and what the virus will be. The hope is that we will be ready and able to develop vaccines against these new viruses with the new pipelines that have been put in place for COVID-19.”
This story was originally published on April 28, 2020, and updated on December 14, 2020.
Read more
FDA votes to approve emergency use of Pfizer coronavirus vaccine
Researchers and volunteers in Rochester have been involved in the testing of the Pfizer/BioNTech vaccine since May, and technologies used in the development of the vaccine can trace their origins to decades of infectious disease research conducted at Rochester.
Bats carry many viruses, including the one behind COVID-19, without becoming ill. University of Rochester biologists are studying the immune system of bats to find potential ways to “mimic” that system in humans.
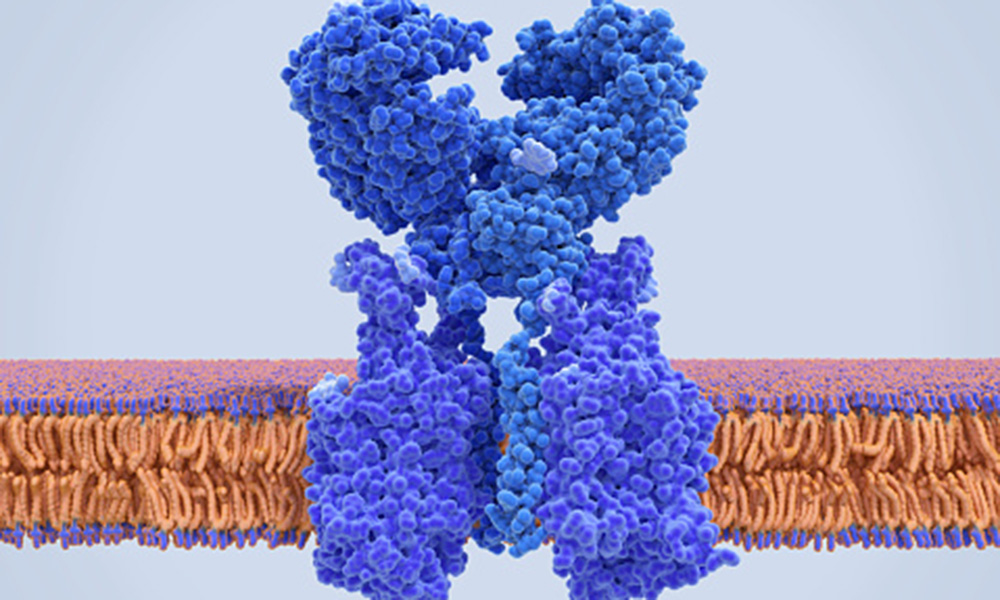
Rochester biologists are exploring how coronavirus interacts with cellular proteins to cause COVID-19 under a priority NSF program.
Category: Science & Technology